Passalacqua Research
Faculty Profile | Research | Teaching | Students | Software
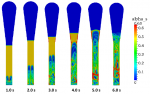
Multiphase computational fluid dynamics
Multiphase flows involving solid particles, droplets or bubbles are involved not only in a variety of industrial and engineering applications, like fluidized bed reactors, fuel atomizers in engines, bubble column and stirred tank chemical reactors, but also in the medical and pharmaceutical industry (drug preparation and delivery), in the transportation industry (i.e. interaction between sand and airplanes, or rain droplets and vehicles), and in environmental science (dispersion of pollutants in the atmosphere). We use advanced continuum models, based on the Gaussian quadrature representation of the probability density function that characterizes the dispersed phase, to describe these types of dispersed multiphase flows, combining accuracy and computational efficiency. These models are capable of simulating complex industrial equipment, describing fine features like the evolution of the particle or bubble size distribution, or predicting particle trajectory crossing.
We generally implement our computational models in open-source codes like OpenFOAM® and MFIX, to facilitate collaborations and dissemination of our developments.
Bubbly flows
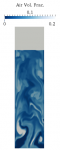
Bubbly flows are often encountered in the chemical and in the oil & gas industry. They are characterized by low Stokes numbers and important variation in the local average bubble diameter. Industrial systems involving bubbly flows are typically non-isothermal, and may operate in conditions where the gas-phase compressibility is not negligible. Depending on the operating conditions, the flow may also be turbulent.
We develop models for these systems that are capable of accounting for the bubble size distribution, the heat transfer phenomena among the phases and the compressibility of the phases. Our models, based on the continuum description of multiphase flows, are capable of predicting the behavior of complex systems including bubble columns, stirred tank reactors and bio-reactors.
Uncertainty quantification
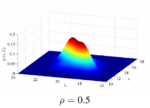
Numerical simulations are affected by uncertainty, exactly as experiments. Uncertainty is present, for example in the information provided to the computational model (input) by the user, and it affects the results of the numerical predictions. It becomes then of paramount importance to develop methods to quantify this uncertainty, and to study how it is propagated from the inputs to the outputs of a computational model. We develop efficient quadrature-based uncertainty quantification methods, based on multi-variate sampling strategies of the probability density function of the values of the uncertain input parameters, and on the direct integration of the moments of the response of the system under examination. Our approach allows the reconstruction of the probability distribution function of the system response to be reconstructed.
We apply our uncertainty quantification method to systems of interest to the energy, oil and chemical/pharmaceutical industry, like fluidized bed reactors, risers, bubble columns and chemical reactors for the preparation of nano-particles.
Numerical methods for computational fluid dynamics
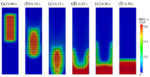
We develop and implement numerical methods for the solution of fluid-dynamic problems, typically concerned with multiphase flow simulations. One of the problems we addressed is the numerical simulation of dense gas-particle flows using Eulerian models, which require robust algorithms capable of dealing with phase separation (absence of one or more phases from one part of the domain), strong phase momentum coupling, and to successfully enforce the maximum physically acceptable value of the particle volume fraction. To achieve this goal, we developed an iterative procedure for the solution of model equations for gas–particle flows and we implemented it into the open-source framework OpenFOAM®, together with strategies to decouple the phase momentum equations, and stabilize the numerical solution.
Funding
- National Science Foundation, Investigation of the lift and virtual mass force in gas-liquid flows with particle-resolved direct numerical simulation, September 2014 – August 2017, Role: PI.
- American Chemical Society – Petroleum Research Fund – Doctoral New Investigator Award, A fundamental investigation of turbulence in multiphase gas-particle flows, September 2014 – August 2016, Role: PI.
- US Department of Energy, Uncertainty quantification tools for multiphase gas-solid flow simulations using MFIX, October 2011 – September 2014, Role: Postdoctoral research associate (2011, 2012, 2013), Co-PI (2013, 2014).